Thyroid
Thyroid gland, its hormones
The thyroid gland consists of two lobes and an isthmus and is located in front of the larynx. The mass of the thyroid gland is 30 g.
The main structural and functional unit of the gland are the follicles - rounded cavities, the wall of which is formed by one row of cells of the cubic epithelium. The follicles are filled with colloid and contain the hormones thyroxin and triiodothyronine, which are associated with thyroglobulin protein. In the interfollicular space are C-cells that produce the hormone thyrocalcitonin. The gland is richly supplied with blood and lymphatic vessels. The amount of blood flowing through the thyroid gland in 1 minute is 3-7 times higher than the mass of the gland itself.
Biosynthesis of thyroxine and triiodothyronine is carried out by iodination of the amino acid tyrosine, therefore, active iodine uptake occurs in the thyroid gland. The iodine content in the follicles is 30 times its concentration in the blood, and with hyperthyroidism, this ratio becomes even greater. Absorption of iodine is carried out by active transport. After tyrosine, which is part of thyroglobulin, is combined with atomic iodine, monoiodotyrosine and diiodotyrosine are formed. Due to the combination of two diiodotyrosine molecules, tetraiodothyronine or thyroxin is formed; condensation of mono- and diiodotyrosine leads to the formation of triiodothyronine. Subsequently, as a result of the action of proteases that cleave thyroglobulin, active hormones are released into the blood.
The activity of thyroxine is several times less than that of triiodothyronine, but the content of thyroxine in the blood is approximately 20 times higher than that of triiodothyronine. When deiodinating, thyroxin can turn into triiodothyronine. Based on these facts, it is suggested that triiodothyronin is the main thyroid hormone, and thyroxin performs the function of its predecessor.
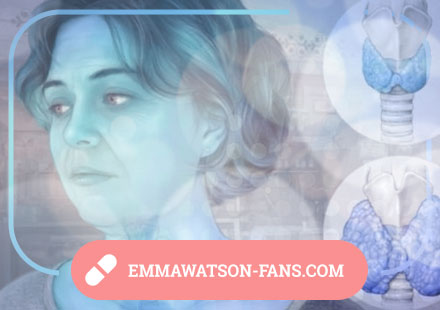
The synthesis of hormones is inextricably linked with the intake of iodine. If there is a deficiency of iodine in the water and soil region, it is also scarce in foods of plant and animal origin. In this case, in order to ensure sufficient synthesis of the hormone, the thyroid gland of children and adults increases in size, sometimes very significantly, i.e. goiter occurs The increase can be not only compensatory, but also pathological, it is called endemic goiter. Lack of iodine in the diet is best compensated by sea kale and other seafood, iodized salt, table mineral water containing iodine, bakery products with iodine additives. However, excessive intake of iodine in the body creates a load on the thyroid gland and can lead to serious consequences.
Thyroid Hormones
Hormone | Characteristic |
Thyroxine (tetraiodothyronine) | A derivative of the amino acid tyrosine, has four atoms of iodine, is synthesized in the follicular tissue |
Triiodothyronine | The amino acid derivative of tyrosine, has three atoms of iodine, is synthesized in the follicular tissue, 4-10 times more active than thyroxin. unstable |
Calcitonin | Polypeptide synthesized in parafollicular tissue and does not contain iodine. |
Effects of thyroxine and triiodothyronine
Main:
- activate the genetic apparatus of the cell, stimulate metabolism, oxygen consumption and intensity of oxidative processes
Metabolic:
- protein metabolism: stimulate protein synthesis, but in the case when the level of hormones exceeds the norm, catabolism prevails;
- fat metabolism: stimulate lipolysis;
- carbohydrate metabolism: during hyperproduction, it stimulates glycogenolysis, blood glucose level rises, activates its entry into the cells, activates liver insulinase
Functional:
- ensure the development and differentiation of tissues, especially nervous;
- enhance the effects of the sympathetic nervous system by increasing the number of adrenoreceptors and inhibition of monoamine oxidase;
- prosimpaticheskih effects are manifested in an increase in heart rate, systolic volume, blood pressure, respiratory rate, intestinal motility, central nervous system excitability, increased body temperature
Manifestations of changes in the production of thyroxine and triiodothyronine
Age | Disadvantage | Excess |
Children | Thyroid Nanism (cretinism) | Basedow's disease (thyrotoxicosis, Graves disease) |
Adults | Myxedema (severe hypothyroidism) | Basedow's disease (thyrotoxicosis, Graves disease) |
Comparative characteristics of insufficient production of somatotropin and thyroxin
Criteria | Pituitary Nanism (dwarfism) | Thyroid Nanism (cretinism) |
Intellectual development | Not broken | Severely depressed |
Childbearing function | Broken | Missing |
Body type | Proportional | Disproportionate |
Effect of thyroid hormones on body functions
The characteristic effect of thyroid hormones (thyroxine and triiodothyronine) is an increase in energy metabolism. The introduction of the hormone is always accompanied by an increase in oxygen consumption, and the removal of the thyroid gland - its decrease. With the introduction of the hormone, the metabolism increases, the amount of energy released increases, the body temperature rises.
Thyroxin increases the consumption of carbohydrates, fats and proteins. There are weight loss and intensive consumption of glucose from the blood by the tissues. The loss of glucose from the blood is compensated by its replenishment due to the enhanced breakdown of glycogen in the liver and muscles. Decreases lipids in the liver, decreases the amount of cholesterol in the blood. Increased excretion of water, calcium and phosphorus.
Thyroid hormones cause anxiety, irritability, insomnia, emotional instability.
Thyroxine increases minute blood volume and heart rate. Thyroid hormone is necessary for ovulation, it contributes to the preservation of pregnancy, regulates the function of the mammary glands.
The growth and development of the body are also regulated by the thyroid gland: a decrease in its function causes growth to stop. Thyroid hormone stimulates blood formation, increases the secretion of the stomach, intestines and milk secretion.
In addition to iodine-containing hormones, thyrocalcitonin is formed in the thyroid gland, which reduces the calcium content in the blood. Calcitonin is an antagonist of the parathyroid hormone of the parathyroid glands. Calcitonin acts on bone tissue, enhances the activity of osteoblasts and the mineralization process. In the kidneys and intestines, the hormone inhibits calcium reabsorption and stimulates the reabsorption of phosphates. The implementation of these effects leads to hypocalcemia.
Hyper and hypofunction of the gland
Hyperfunction (hyperthyroidism) is the cause of the disease, called basilial disease. The main symptoms of the disease are goitre, puze-eyed, increased metabolism, heart rate, increased sweating, motor activity (fussiness), irritability (moodiness, rapid mood swings, emotional instability), fatigue. Goiter is formed by a diffuse enlargement of the thyroid gland. Now methods of treatment are so effective that severe cases of the disease are quite rare.
Hypofunction (hypothyroidism) of the thyroid gland, which occurs at an early age, up to 3-4 years, causes the development of symptoms of cretinism. Children suffering from cretinism are lagging behind in physical and mental development. Symptoms of the disease: dwarf growth and disturbance of body proportions, a wide, deep-rooted nose bridge, wide-open eyes, an open mouth and a constantly protruding tongue, as it does not interfere with the mouth, short and curved limbs, dull facial expression. The life expectancy of such people usually does not exceed 30-40 years. In the first 2-3 months of life, you can achieve subsequent normal mental development. If treatment begins at the age of one year, then 40% of children who have been exposed to this disease remain at a very low level of mental development.
Hypofunction of the thyroid gland in adults leads to the occurrence of a disease called myxedema, or mucous edema. In this disease, the intensity of metabolic processes decreases (by 15-40%), body temperature, pulse becomes less frequent, blood pressure decreases, puffiness appears, hair falls out, nails break, face becomes pale, lifeless, mask-like. Patients are slow, drowsy, poor memory. Myxedema is a slowly progressive disease that, if left untreated, leads to complete disability.
Regulation of thyroid function
A specific regulator of the activity of the thyroid gland is iodine, the thyroid hormone itself and TSH (thyroid stimulating hormone). Iodine in small doses increases the secretion of TSH, and in large doses inhibits it. The thyroid gland is controlled by the CNS. Such foods as cabbage, swede, turnip, inhibit the function of the thyroid gland. The production of thyroxine and triiodothyronine is greatly enhanced under conditions of prolonged emotional arousal. It is also noted that the secretion of these hormones is accelerated with a decrease in body temperature.
Manifestations of disorders of the endocrine function of the thyroid gland
With an increase in the functional activity of the thyroid gland and excessive production of thyroid hormones, a state of hyperthyroidism (hyperthyroidism) occurs, which is characterized by an increase in the blood level of thyroid hormones. Manifestations of this condition are explained by the effects of tirsoid hormones in elevated concentrations. Thus, due to an increase in basal metabolic rate (hypermetabolism), a slight increase in body temperature (hyperthermia) is observed in patients. Body weight is reduced despite maintaining or increasing appetite. This condition is manifested by an increase in oxygen demand, tachycardia, an increase in myocardial contractility, an increase in systolic blood pressure, and an increase in pulmonary ventilation. The activity of the PCA increases, the number of p-adrenorecenters increases, sweating and intolerance to heat develop. Anxiety and emotional lability increase, limb tremor and other changes in the body may appear.
Increased formation and secretion of thyroid hormones can cause a number of factors, the choice of a method for correcting thyroid function depends on the correct detection. Among them are factors that cause hyperfunction of the follicular cells of the thyroid gland (tumors of the gland, mutation of G-proteins) and an increase in the formation and secretion of thyroid hormones. Thyrocyte hyperfunction is observed with excessive stimulation of thyrotropin receptors with increased levels of TSH, for example, with pituitary tumors, or reduced sensitivity of thyroid hormone receptors in thyroid trophic adenohypophysis. A frequent cause of thyrocyte hyperfunction, an increase in the size of the gland is the stimulation of the TSH receptors by antibodies produced to them in an autoimmune disease called Graves-Basedow's disease (Fig. 1). A temporary increase in the level of thyrsoid hormones in the blood can develop when thyrocyte is destroyed due to inflammatory processes in the gland (Hashimoto toxic thyroiditis), and excessive amounts of thyroid hormones and iodine preparations are taken.
An increase in thyroid hormone levels may manifest as thyrotoxicosis; in this case, they speak of hyperthyroidism with thyrotoxicosis. But thyrotoxicosis can develop when an excess amount of thyroid hormones is introduced into the body, in the absence of hyperthyroidism. The development of thyrotoxicosis due to the increased sensitivity of cell receptors to thyroid hormones is described. There are also opposite cases in which the sensitivity of cells to thyroid hormones is reduced and a state of resistance to thyroid hormones develops.
The reduced formation and secretion of thyroid hormones can be caused by many reasons, some of which are the result of dysfunction of the mechanisms regulating the function of the thyroid gland. Thus, hypothyroidism (hypothyroidism) can develop by reducing the formation of TRH in the hypothalamus (tumors, cysts, radiation, encephalitis in the hypothalamus, etc.). Such hypothyroidism is called tertiary. Secondary hypothyroidism develops due to insufficient formation of THG by the pituitary gland (tumors, cysts, radiation, surgical removal of part of the pituitary, encephalitis, etc.). Primary hypothyroidism can develop due to autoimmune inflammation of the gland, with iodine deficiency, selenium, excessive intake of goitrogenic products - goitrogens (some cabbage varieties), after irradiation of the gland, long-term use of a number of drugs (iodine, lithium preparations, antithyroid drugs), etc.
Insufficient production of thyroid hormones leads to a decrease in the intensity of metabolism, oxygen consumption, ventilation, myocardial contractility, and minute blood volume. In severe hypothyroidism, a condition called myxedema - mucous edema can develop. It develops due to the accumulation (possibly under the influence of elevated TSH levels) of mucopolysaccharides and water in the basal layers of the skin, which leads to puffiness of the face and doughy consistency of the skin, as well as an increase in body weight, despite a decrease in appetite. Patients with myxedema can develop mental and motor inhibition, drowsiness, chilliness, reduced intelligence, tone of the sympathetic section of the ANS, and other changes.
In the implementation of complex processes of formation of thyroid hormones involved ion pumps, providing iodine, a number of enzymes of protein nature, among which the key role is played by thyroperoxidase. In some cases, a person may have a genetic defect leading to a violation of their structure and function, which is accompanied by a violation of the synthesis of thyroid hormones. There may be genetic defects in thyroglobulin structure. Against thyroperoxidase and thyroglobulin, autoantibodies are often produced, which is also accompanied by a violation of the synthesis of thyroid hormones. The activity of the capture of iodine and its inclusion in the composition of thyroglobulin can be influenced by a number of pharmacological agents, regulating the synthesis of hormones. Pa their synthesis can be influenced by taking iodine preparations.
The development of hypothyroidism in the fetus and newborns can lead to the appearance of cretinism - physical (short stature, disruption of body proportions), sexual and mental underdevelopment. These changes can be prevented with adequate thyroid hormone replacement therapy in the first months after the baby is born.
Thyroid structure
The thyroid gland is by its mass and size the largest endocrine organ. It usually consists of two lobes connected by an isthmus, and is located on the front surface of the neck, being fixed to the front and side surfaces of the trachea and larynx with connective tissue. The average weight of a normal thyroid gland in adults ranges from 15-30 g, but its size, shape and topography of the location vary widely.
The functionally active thyroid gland of the first of the endocrine glands appears in the process of embryogenesis. The laying of the thyroid gland in a human fetus is formed on the 16th-17th day of intrauterine development in the form of a cluster of endodermal cells at the root of the tongue.
In the early stages of development (6-8 weeks), the anlage of the gland is a layer of intensely proliferating epithelial cells. During this period there is a rapid growth of the gland, but hormones are not yet formed in it. The first signs of their secretion are detected in the 10-11th week (in fetuses about 7 cm in size), when the gland cells are able to absorb iodine, form a colloid and synthesize thyroxin.
Single follicles appear in the capsule, in which follicular cells are formed.
Parafollicular (near follicular) or C-cells grow into the anlage of the thyroid gland from the 5th pair of gill pockets. By the 12th and 14th weeks of fetal development, the entire right lobe of the thyroid gland acquires a follicular structure, and the left - two weeks later. By the 16th to 17th weeks, the fetal thyroid gland is already fully differentiated. Thyroid glands of fetuses 21-32 weeks of age are characterized by high functional activity, which continues to grow up to 33-35 weeks.
In the parenchyma of the gland, three types of cells are distinguished: A, B, and C. The bulk of the parenchyma cells are thyrocytes (follicular or A-cells). They line the wall of the follicles, in the cavities of which the colloid is located. Each follicle is surrounded by a dense network of capillaries, into the lumen of which thyroxin and triiodothyronine secreted by the thyroid gland are absorbed.
In the unchanged thyroid gland, the follicles are evenly distributed throughout the parenchyma. With a low functional activity of the gland, thyrocytes are usually flat, with high - cylindrical (the height of the cells is proportional to the degree of activity of the processes carried out in them). The colloid that fills the follicle lumen is a homogeneous, viscous fluid. The bulk of the colloid is thyreoglobulin secreted by thyrocytes into the lumen of the follicle.
B-cells (Ashkenazi-Gyurtl cells) are larger than thyrocytes, have eosinophilic cytoplasm and a rounded centrally located nucleus. Biogenic amines, including serotonin, are found in the cytoplasm of these cells. For the first time, B cells appear between the ages of 14-16. In large numbers, they are found in people aged 50-60 years.
Parafollicular, or C-cells (in Russian transcription of K-cells), differ from thyrocytes in their lack of ability to absorb iodine. They provide the synthesis of calcitonin - a hormone involved in the regulation of calcium metabolism in the body. C-cells are larger than thyrocytes, as a rule, in the follicles are located singly. Their morphology is characteristic of cells that synthesize protein for export (there is a rough endoplasmic reticulum, Golgi complex, secretory granules, mitochondria). On histological specimens, the cytoplasm of C-cells looks lighter than the cytoplasm of thyrocytes, hence their name - light cells.
If at the tissue level the main structural and functional unit of the thyroid gland are the follicles surrounded by basal membranes, one of the supposed organ units of the thyroid gland can be micro-segments, which include follicles, C-cells, hemocapillaries, tissue basophils. The microbubs include 4-6 follicles surrounded by a fibroblast membrane.
By the time of birth, the thyroid gland is functionally active and structurally fully differentiated. In newborns, follicles are small (60–70 µm in diameter), as the child’s body develops, their size increases and reaches 250 µm in adults. In the first two weeks after birth, the follicles develop intensively, by 6 months they are well developed throughout the gland, and by the year reach a diameter of 100 microns. During puberty, there is an increase in the growth of the parenchyma and stroma of the gland, an increase in its functional activity, manifested by an increase in the height of thyrocytes, and an increase in the activity of enzymes in them.
In an adult, the thyroid gland is adjacent to the larynx and the upper part of the trachea in such a way that the isthmus is located at level II-IV of the tracheal half-rings.
The mass and size of the thyroid gland changes throughout life. In a healthy newborn, the mass of the gland varies from 1.5 to 2 g. By the end of the first year of life, the mass doubles and slowly increases by the period of puberty to 10-14 g. The increase in mass is especially noticeable at the age of 5-7 years. The mass of the thyroid gland at the age of 20-60 years ranges from 17 to 40 g.
The thyroid gland has an exceptionally abundant blood supply compared with other organs. The volumetric rate of blood flow in the thyroid gland is about 5 ml / g per minute.
The thyroid gland is supplied by the paired upper and lower thyroid arteries. Sometimes the unpaired, lowest artery (a. Thyroidea ima) participates in the blood supply.
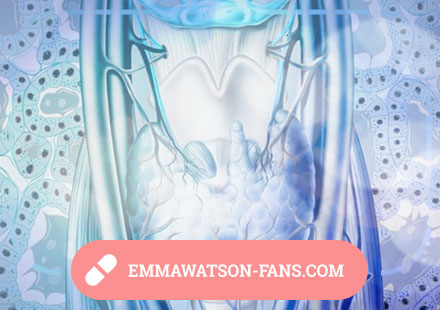
Outflow of venous blood from the thyroid gland is carried out through the veins forming the plexus in the circumference of the side lobes and the isthmus. The thyroid gland has an extensive network of lymphatic vessels, through which the lymph takes care of the deep cervical lymph nodes, then the supraclavicular and lateral cervical deep lymph nodes. On each side of the neck, the carrying lymphatic vessels of the lateral cervical deep lymph nodes form a jugular trunk, which flows into the left thoracic duct and to the right into the right lymphatic duct.
The thyroid gland is innervated by the postganglionic fibers of the sympathetic nervous system from the upper, middle (mainly) and lower cervical nodes of the sympathetic trunk. Thyroid nerves form plexuses around vessels suitable for the gland. It is believed that these nerves perform vasomotor function. The vagus nerve, which carries parasympathetic fibers to the gland as part of the upper and lower laryngeal nerves, is also involved in the innervation of the thyroid gland. Synthesis of iodine-containing thyroid hormones T3 and T4 is carried out by follicular A-cells-thyrocytes. Hormones T3 and T4 are iodized.
The hormones T4 and T3 are iodinated derivatives of the amino acid L-tyrosine. Iodine, which is part of their structure, makes up 59-65% of the mass of the hormone molecule. The need for iodine for the normal synthesis of thyroid hormones is presented in Table. 1. The sequence of synthesis processes is simplified as follows. Iodine in the form of iodide is captured from the blood using an ion pump, accumulates in thyrocytes, is oxidized and incorporated into the phenolic ring of tyrosine in the composition of thyroglobulin (the organization of iodine). Iodization of thyroglobulin with the formation of mono- and diiodotyrosines occurs at the border between thyrocyte and colloid. Next is the connection (condensation) of two diiodotyrosine molecules with the formation of T4 or diiodotyrosine and monoiodotyrosine with the formation of T3. Part of thyroxine is deiodinated in the thyroid gland to form triiodothyronine.
Table 1. Iodine intake rates (WHO, 2005. by I. Dedov et al. 2007)
Groups of people | The need for iodine µg / day |
Preschool children (from 0 to 59 months) | 90 |
School children (from 6 to 12 years) | 120 |
Teens and adults (over 12 years old) | 150 |
Pregnant women and women during breastfeeding | 250 |
Iodinated thyroglobulin together with T4 and T3 attached to it accumulates and is stored in the follicles as a colloid, acting as depot-thyroid hormones. The release of hormones occurs as a result of pinocytosis of the follicular colloid and the subsequent hydrolysis of thyroglobulin in phagolysosomes. The released T4 and T3 are secreted into the blood.
The basal daily secretion of the thyroid gland is about 80 µg T4 and 4 µg T3. In this case, the thyrocytes of the thyroid follicles are the only source of endogenous T4 formation. In contrast to T4, T3 is formed in thyrocytes in a small amount, and the main formation of this active form of the hormone is carried out in the cells of all tissues of the body by deiodinating about 80% of T4.
Normally, the content of T4 in the blood is 60-160 nmol / l, and T3 - 1-3 nmol / l. The half-life of T4 is about 7 days, and T3 is 17-36 hours. Both hormones are hydrophobic, 99.97% of T4 and 99.70% of T3 are transported by blood in bound form with plasma proteins - thyroxin-binding globulin, prealbumin and albumin.
Thus, in addition to the glandular depot of thyroid hormones in the body there is a second - an extra-iron depot of thyroid hormones, represented by hormones associated with transport proteins of the blood. The role of these depots is to prevent the rapid decrease in the level of thyroid hormones in the body, which could occur with a short-term decrease in their synthesis, for example, with a brief decrease in iodine intake. The bound form of hormones in the blood prevents their rapid elimination from the body through the kidneys, protects cells from the uncontrolled intake of hormones in them. The cells receive free hormones in amounts commensurate with their functional needs.
Thyroxin entering cells is deiodinated by the action of deiodinase enzymes, and when one atom of iodine is removed, a more active hormone, triiodothyronine, is formed from it. In this case, depending on the paths of deiodination, both active T3 and inactive reverse T3 (3,3 ', 5'-triiod-L-thyronin - pT3) can be formed from T4. These hormones, by successive deiodination, are converted to T2 metabolites, then T1 and T0, which are conjugated with glucuronic acid or sulfate in the liver and excreted in the bile and via the kidneys from the body. Not only T3, but other metabolites of thyroxine can also exhibit biological activity.
The mechanism of action of tirsoid hormones is primarily due to their interaction with nuclear receptors, which are non-histone proteins located directly in the nucleus of cells. There are three main subtypes of thyrcid hormone receptors: TPβ-2, TPβ-1 and TPa-1. As a result of interaction with the T3 receptor is activated, the hormone receptor complex interacts with the hormone-sensitive DNA region and regulates the transcriptional activity of genes.
A number of non-genomic effects of thirsoid hormones in the mitochondria, the plasma membrane of cells, have been identified. In particular, thyroid hormones can change the permeability of mitochondrial membranes for hydrogen protons and, by separating the processes of respiration and phosphorylation, reduce ATP synthesis and increase the formation of heat in the body. They change the permeability of plasma membranes for Ca2 + ions and have an impact on many intracellular processes carried out with the participation of calcium.
Main effects and role of thyroid hormones
Normal functioning of all organs and tissues of the body without exception is possible at normal levels of thyroid hormones, as they affect the growth and maturation of tissues, energy exchange and the exchange of proteins, lipids, carbohydrates, nucleic acids, vitamins and other substances. The metabolic and other physiological effects of thyroid hormones are excreted.
Metabolic effects:
- activation of oxidative processes and an increase in basal metabolism, increased absorption of oxygen by the tissues, increased heat generation and body temperature;
- stimulation of protein synthesis (anabolic effect) in physiological concentrations;
- increased fatty acid oxidation and lower blood levels;
- hyperglycemia due to the activation of glycogenolysis in the liver.
Physiological effects:
- ensuring the normal processes of growth, development, differentiation of cells, tissues and organs, including the central nervous system (myelination of nerve fibers, differentiation of neurons), as well as processes of physiological tissue regeneration;
- enhancing the effects of SNA through increasing the sensitivity of adrenoreceptors to the action of Adr and ON;
- increasing the excitability of the central nervous system and activation of mental processes;
- participation in ensuring reproductive function (contribute to the synthesis of GH, FSH, LH and the implementation of the effects of insulin-like growth factor - IGF);
- participation in the formation of adaptive reactions of the body to adverse effects, in particular, cold;
- participation in the development of the muscular system, an increase in the strength and speed of muscle contractions.
The regulation of the formation, secretion and transformations of thyroid hormones is carried out by complex hormonal, nervous and other mechanisms. Their knowledge allows you to diagnose the causes of reduced or increased secretion of thyroid hormones.
The hormones of the hypothalamic-pituitary-thyroid axis play a key role in the regulation of the secretion of thyroid hormones. Basal secretion of thyroid hormones and its changes during various influences is regulated by the level of hypothalamus TRH and pituitary TSH. TRG stimulates the production of TSH, which has a stimulating effect on almost all processes in the thyroid gland and secretion of T4 and T3. Under normal physiological conditions, the formation of TRH and TSH are controlled by the level of free T4 and T. in the blood based on negative feedback mechanisms. At the same time, the secretion of TRG and TSH is inhibited by a high level of thyroid hormones in the blood, and at their low concentration increases.
Important in the mechanisms of regulation of hormones of the hypothalamic-pituitary-thyroid axis is the state of sensitivity of the receptors to the action of hormones at different levels of the axis. Changes in the structure of these receptors or their stimulation with autoantibodies can be the causes of impaired formation of thyroid hormones.
The formation of hormones in the gland itself depends on the receipt of a sufficient amount of iodide from the blood - 1-2 mg per 1 kg of body weight.
With insufficient intake of iodine in the body, adaptation processes develop in it, which are aimed at the most careful and efficient use of iodine present in it. They consist in enhancing blood flow through the gland, more efficiently capturing iodine from the blood of the thyroid gland, changing the processes of hormone synthesis and secretion. Adaptive reactions are triggered and regulated by thyrotropin, the level of which increases with iodine deficiency. If the daily intake of iodine in the body is less than 20 micrograms for a long time, then long-term stimulation of thyroid cells leads to the growth of its tissue and the development of goiter.
Self-regulating mechanisms of the gland in conditions of iodine deficiency provide its greater capture by thyrocytes with a lower level of iodine in the blood and more effective reutilization. If about 50 mcg of iodine is delivered per day to the body, then due to an increase in the rate of its absorption by thyrocytes from the blood (food-grade iodine and recycled iodine from metabolic products), about 100 mcg of iodine enters the thyroid gland per day.
The intake of 50 µg iodine per day from the gastrointestinal tract is the threshold at which the long-term ability of the thyroid gland to accumulate it (including recycled iodine) in quantities remains when the content of inorganic iodine in the gland remains at the lower limit of normal (about 10 mg). Below this threshold intake of iodine in the body per day, the effectiveness of the increased capture rate of iodine by the thyroid gland is insufficient, the absorption of iodine and its content in the gland decreases. In these cases, the development of thyroid dysfunction becomes more likely.
Simultaneously with the inclusion of the adaptation mechanisms of the thyroid gland with iodine deficiency, a decrease in its excretion from the body with the urine is observed. As a result, adaptive excretory mechanisms provide the excretion of iodine per day in amounts equivalent to its lower daily intake from the gastrointestinal tract.
The intake of subliminal iodine concentrations (less than 50 μg per day) leads to an increase in TSH secretion and its stimulating effect on the thyroid gland. This is accompanied by an acceleration of iodination of tyrosyl residues of thyroglobulin, an increase in the content of monoiodine nucleosides (MIT) and a decrease in diiodotyrosines (DIT). The MIT / DIT ratio increases, and, as a result, T4 synthesis decreases and T3 synthesis increases. The ratio T3 / T4 increases in gland and blood.
In severe iodine deficiency, there is a decrease in serum T4 levels, an increase in the level of TSH and a normal or elevated T3 content. The mechanisms of these changes are not precisely elucidated, but most likely this is a result of an increase in the rate of formation and secretion of T3, an increase in the ratio of T3T4 and an increase in the conversion of T4 to T3 in peripheral tissues.
The increase in the formation of T3 in the conditions of iodine deficiency is justified from the point of view of achieving the greatest final metabolic effects of TG with the least of their “iodine” capacity. It is known that the effect on T3 metabolism is about 3-8 times stronger than T4, but since T3 contains only 3 iodine atoms in its structure (and not 4 as T4), only 75% iodine is needed for the synthesis of one T3 molecule costs, compared with the synthesis of T4.
With a very significant iodine deficiency and a decrease in the function of the thyroid gland against the background of a high level of TSH, the levels of T4 and T3 decrease. In serum, more thyroglobulin appears, the level of which correlates with the level of TSH.
Iodine deficiency in children has a stronger effect than on adults on the metabolism processes in thyroid thyroid cells. In iodine-deficient areas of residence, thyroid dysfunction in newborns and children is much more common and more pronounced than in adults.
When a small excess of iodine enters the human body, the degree of organization of iodide increases, the synthesis of TG and their secretion. There is an increase in the level of TSH, a slight decrease in the level of free T4 in serum while simultaneously increasing the content of thyroglobulin in it. Longer excess iodine intake can block the synthesis of TG by inhibiting the activity of enzymes involved in biosynthetic processes. By the end of the first month an increase in the size of the thyroid gland is noted. With chronic excess intake of excess iodine in the body, hypothyroidism can develop, but if the intake of iodine in the body has normalized, then the size and function of the thyroid gland can return to their original values.
Sources of iodine, which can be the cause of its excessive intake, are often iodized salt, complex multivitamin preparations containing mineral supplements, foods, and some iodine-containing drugs.
The thyroid gland has an internal regulatory mechanism that allows you to effectively deal with excess iodine intake. Although iodine intake in the body can fluctuate, the concentration of TG and TSH in the serum may remain unchanged.
It is believed that the maximum amount of iodine, which when injected into the body does not cause changes in thyroid function, is about 500 mcg per day for adults, but an increase in the level of TSH secretion to the action of thyrotropin-releasing hormone is observed.
The intake of iodine in quantities of 1.5-4.5 mg per day leads to a significant decrease in serum levels, both total and free T4, an increase in the level of TSH (the level of T3 remains unchanged).
The effect of suppressing thyroid function with excess iodine also occurs in thyrotoxicosis, when, by taking an excessive amount of iodine (relative to the natural daily need), the symptoms of thyrotoxicosis are eliminated and the serum TG level is lowered. However, with prolonged intake of excess iodine, thyrotoxicosis manifestations return again. It is believed that a temporary decrease in the level of TG in the blood with an excessive intake of iodine is primarily due to the inhibition of the secretion of hormones.
The intake of small excess iodine leads to a proportional increase in its uptake by the thyroid gland, to some saturating value of absorbed iodine. When this value is reached, the capture of iodine by the gland can decrease despite its intake into the body in large quantities. Under these conditions, under the influence of the pituitary TSH, the activity of the thyroid gland can vary widely.
Since the intake of excess iodine in the body increases the level of TSH, we should not expect an initial suppression, but an activation of the function of the thyroid gland. However, it has been established that iodine inhibits an increase in adenylate cyclase activity, inhibits the synthesis of thyroperoxidase, inhibits the formation of hydrogen peroxide in response to the action of TSH, although the binding of TSH to the receptor of the thyrocyte cell membrane is not violated.
It has already been noted that the suppression of thyroid function by excess iodine is temporary and soon the function is restored despite the continued intake of excess iodine in the body. There is an adaptation or escape of the thyroid gland from the influence of iodine. One of the main mechanisms of this adaptation is to reduce the efficiency of capture and transport of iodine to thyrocyte. Since it is believed that the transport of iodine through the basement membrane of the thyrocyte is associated with the function of Na + / K + ATPase, it can be expected that an excess of iodine may affect its properties.
Despite the existence of mechanisms of adaptation of the thyroid gland to insufficient or excessive intake of iodine to maintain its normal function in the body, iodine balance must be maintained. With a normal level of iodine in the soil and water per day, up to 500 μg of iodine in the form of iodide or iodate can be supplied to the human body with plant foods and to a lesser extent with water, which turn into iodides in the stomach. Iodides are rapidly absorbed from the gastrointestinal tract and are distributed in the extracellular body fluid. The concentration of iodide in the extracellular spaces remains low, as part of the iodide is quickly captured from the extracellular fluid by the thyroid gland, and the rest is excreted from the body at night. The rate of capture of iodine by the thyroid gland is inversely proportional to the rate of its excretion by the kidneys. Iodine can be excreted by the salivary and other glands of the digestive tract, but then reabsorbed again from the intestine into the blood. About 1-2% of iodine is excreted by the sweat glands, and with increased sweating, the proportion of iodine excreted with iodine can reach 10%.
Of the 500 μg of iodine absorbed from the upper intestine into the blood, about 115 μg is captured by the thyroid gland and about 75 μg of iodine is used per day for TG synthesis, 40 μg is returned back to the extracellular fluid. Synthesized T4 and T3 are subsequently destroyed in the liver and other tissues, iodine released in an amount of 60 μg enters the blood and extracellular fluid, and about 15 μg of iodine conjugated in the liver with glucuronides or sulphates are removed as part of bile.
In the total volume, blood is an extracellular fluid, which constitutes about 35% of body weight (or about 25 liters) in an adult, in which about 150 μg iodine is dissolved. Iodide is freely filtered in the glomeruli and approximately 70% passively reabsorbed in the tubules. During the day, about 485 mcg of iodine is excreted in the urine and about 15 mcg - with feces. The average concentration of iodine in the blood plasma is maintained at about 0.3 μg / l.
By reducing the intake of iodine in the body, its amount in body fluids decreases, urinary excretion decreases, and the thyroid gland can increase its absorption by 80-90%. The thyroid gland is able to store iodine in the form of iodothyronines and iodinated tyrosines in quantities close to the 100-day need of the body. Due to these iodine-saving mechanisms and deposited iodine, TG synthesis under conditions of iodine deficiency in the body can remain intact for a period of up to two months. Longer iodine deficiency in the body leads to a decrease in the synthesis of TG despite its maximum seizure of blood from the gland. An increase in iodine intake can accelerate TG synthesis. However, if the daily intake of iodine exceeds 2000 µg, the accumulation of iodine in the thyroid gland reaches a level when iodine uptake and hormone biosynthesis are inhibited. Chronic iodine intoxication occurs when its daily intake in the body is more than 20 times the daily requirement.
Iodide entering the body is excreted mainly from the urine, so its total content in the volume of daily urine is the most accurate indicator of iodine intake and can be used to estimate the iodine balance in the whole organism.
Thus, a sufficient intake of exogenous iodine is necessary for the synthesis of TG in quantities that are adequate to the needs of the body. At the same time, the normal realization of the effects of TG depends on the efficiency of their binding to the nuclear receptors of cells containing zinc. Consequently, the intake of a sufficient amount of this trace element (15 mg / day) is also important for the manifestation of the effects of TG at the level of the cell nucleus.
The formation in the peripheral tissues of the active forms of TG from thyroxin occurs under the action of deiodinases, for the manifestation of the activity of which the presence of selenium is necessary. It has been established that intake of selenium in quantities of 55-70 mcg per day in an adult human body is a necessary condition for the formation of a sufficient amount of Tv in peripheral tissues.
The nervous mechanisms regulating the function of the thyroid gland are carried out through the influence of neurotransmitters ATP and PSNS. SNA innervates the glandular vessels and glandular tissue with its postganglionic fibers. Noradrenaline increases the level of cAMP in thyrocytes, enhances their absorption of iodine, the synthesis and secretion of thyroid hormones. PSN fibers are also suitable for the follicles and vessels of the thyroid gland. An increase in PSN tone (or the introduction of acetylcholine) is accompanied by an increase in the level of cGMP in thyrocytes and a decrease in the secretion of thyroid hormones.
Under the control of the central nervous system is the formation and secretion of TRG small cell neurons of the hypothalamus, and therefore, the secretion of TSH and thyroid hormones.
The level of thyroid hormones in the cells of tissues, their transformation into active forms and metabolites is regulated by the system of deiodinases - enzymes whose activity depends on the presence of selenocysteine in the cells and the intake of selenium. There are three types of deiodinases (D1, D2, DZ), which are differently distributed in different tissues of the body and determine the ways of conversion of thyroxin into active T3, or inactive pT3 and other metabolites.
Endocrine function of parafollicular thyroid K-cells
These cells synthesize and secrete the hormone calcitonin.
Calcitonip (thyrecalcitoiin) is a peptide consisting of 32 amino acid residues, the blood content is 5-28 pmol / l, acts on target cells, stimulating T-TMS-membrane receptors and increasing the level of cAMP and IHP in them. It can be synthesized in the thymus, lungs, central nervous system and other organs. The role of extra thyroid calcitonin is unknown.
The physiological role of calcitonin is the regulation of the level of calcium (Ca2 +) and phosphate (PO34-) in the blood. The function is implemented through several mechanisms:
- inhibition of the functional activity of osteoclasts and suppression of bone resorption. This reduces the excretion of Ca2 + and PO34-ions from the bone tissue into the blood;
- reducing the reabsorption of Ca2 + and PO34 ions from primary urine in the renal tubules.
Due to these effects, an increase in calcitonin level leads to a decrease in the content of Ca2 and PO34 ions in the blood.
Regulation of calcitonin secretion is carried out with the direct participation of Ca2 in the blood, the concentration of which normally is 2.25-2.75 mmol / l (9-11 mg%). Increasing the level of calcium in the blood (gypsrcalcism) causes active secretion of calcitonin. A decrease in calcium levels leads to a decrease in secretion of the hormone. Stimulate calcitonin secretion by catecholamines, glucagon, gastrin and cholecystokinin.
An increase in the level of calcitonin (50–5,000 times higher than the norm) is observed in one of the forms of thyroid cancer (medullary carcinoma), which develops from parafollicular cells. Moreover, the determination of high levels of calcitonin in the blood is one of the markers of this disease.
Increasing the level of calcitonin in the blood, as well as the almost complete absence of calcitonin after the removal of the thyroid gland, may not be accompanied by impaired calcium metabolism and the state of the skeletal system. These clinical observations suggest that the physiological role of calcitonin in regulating calcium levels is not fully understood.